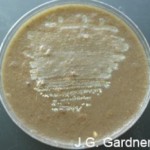
The degradation of polysaccharides is critical in a variety of contexts, specifically environmental, biomedical, and industrial. For example, the complex polysaccharides that comprise plant cell walls, fungal cell walls, or crustacean shells are abundant terrestrial and marine sources of carbon for the growth of microorganisms. For example, a calculated 100 billion tons of plant polymers are turned over every year as an essential arc in the carbon cycle. Microbial activities in both terrestrial and marine environments are the main driver of this turnover. In addition, the microbial flora in human digestive tracts contributes nutritionally with the breakdown of the plant-derived polysaccharides eaten. It is estimated that 10% of your daily caloric intake comes from the short chain fatty acids that are the byproducts of cellulose degradation in your gut. Finally, polysaccharide degradation is critical biotechnologically because it is currently one of the more expensive parts of biofuel production. If low-cost biofuels are to compete with fossil fuels, we need to understand how these polymers are efficiently degraded.
The polysaccharides found in plant cell walls, fungal cell walls, and crustacean shells are difficult to degrade (recalcitrant), and this is due to the structural and chemical complexity of the polymers, in addition to the overall insolubility of these polymers. Collectively these traits prevent efficient depolymerization and subsequent sugar utilization of polysaccharides by the vast majority of microorganisms in the environment.
Research in the Gardner laboratory uses an interdisciplinary approach including systems biology (transcriptomics and proteomics), classical bacterial genetics (targeted and random gene disruptions), and biochemistry (enzyme purification and assay) to understand the regulation and mechanisms of recalcitrant polysaccharide degradation by bacteria. We use the bacterium Cellvibrio japonicus because of the sophisticated genetic tools to manipulate the microorganism, and because this bacterium has the incredible ability to completely depolymerize both plant cell wall, fungal cell wall, and crustacean shell polysaccharides to obtain carbon and energy. We have four main questions that drive our research:
(1) How do bacteria sense the environment and detect recalcitrant polysaccharides?
The crystalline and hydrophobic nature of the polymers that comprise plant cell wall, fungal cell wall, and crustacean shell polysaccharides make them largely insoluble and inaccessible to most bacteria. Our transcriptomic data shows that C. japonicus is not only able to detect these polymers, but can alter the composition of degradative enzymes produced over time as it depolymerizes the substrate. Currently, our lab is dissecting what signals cause these physiological and metabolic changes necessary for insoluble polysaccharide degradation.
(2) What proteins are needed for complete consumption of recalcitrant polysaccharides?
There are several hundred genes predicted to be involved in degrading recalcitrant polysaccharides in C. japonicus. However, it is unclear which genes encode proteins with functions that are absolutely critical for degradation. Work in our lab has identified several gene products that are essential for the degradation of cellulose, xylan, xyloglucan, chitin, and trehalose. Through mutational analysis and enzymatic assay we are expanding our understanding of what proteins are essential for plant cell wall, fungal cell wall, and crustacean shell polysaccharide degradation by C. japonicus.
(3) How is the process of recalcitrant polysaccharide detection, degradation, and consumption regulated?
With several hundred proteins to synthesize and export to degrade recalcitrant polysaccharides, it is unclear how this process is regulated. Our transcriptomic and genetic data suggest that degradation is done in a coordinated manner, and we have identified several pathways critical for degradation. Further analysis of the physiological and metabolic changes that take place during the course of recalcitrant polysaccharide degradation will elucidate how this complex process unfolds. We have begun to use computational biology and bioinformatic approaches to identify suites of co-regulated genes.
(4) How can we use our understanding of recalcitrant polysaccharides degradation for industrial or medical applications?
We have previously engineered an ethanol production pathway into C. japonicus and shown that when grown on cellulose, C. japonicus can produce bio-ethanol. Current work in our lab uses synthetic biology approaches to examine the possibility of incorporating novel metabolic pathways into bacteria with useful industrial or biomedical properties. Examples of current work are the synthesis of value added chemicals and developing treatments for fungal diseases using bacterial enzymes.